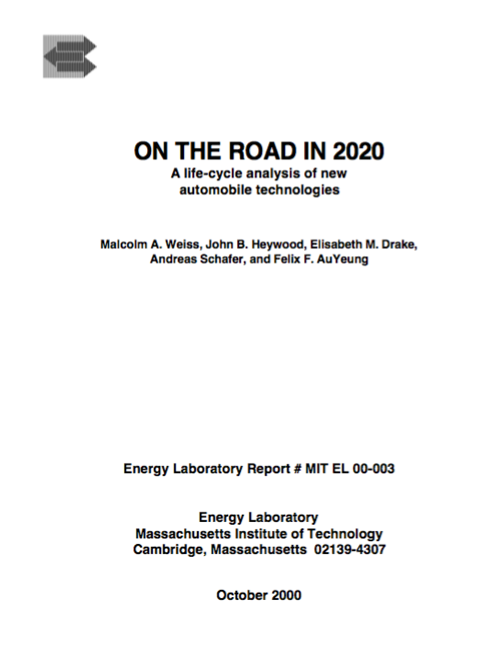
Abstract
This report is a description of work done at MIT during the past two years to assess technologies for new passenger cars that could be developed and commercialized by the year 2020. The report does not make predictions about which technologies will be developed nor judgments about which technologies should be developed issues for the marketplace and for public policy that are not examined here.
The primary motivation for this study was the desire to assess new automobile technologies which have the potential to function with lower emissions of greenhouse gases (GHGs) widely believed to contribute to global warming. The GHG of most concern here is carbon dioxide (CO2), but methane (CH4) and nitrous oxide (N2O) can also be important. If public policy or market forces result in constraints on GHG emissions, automobiles and other light- duty vehicles a key part of the transportation sector will be candidates for those constraints since the transportation sector accounts for about 30% of all CO2 emissions in OECD countries, and about 20% worldwide.
Methodology
To assess and compare future technologies validly, the methodology must include three main elements:
- Assessment of the total system over its entire life cycle.
- Assessment of all the important characteristics of the technology at the same future date.
- Assessment of the impacts of each of those characteristics and transitional changes on each of the main stakeholder groups.
The life cycle of automotive technology is defined here to include all the steps required to provide the fuel, to manufacture the vehicle, and to operate and maintain the vehicle throughout its lifetime up to scrappage and recycling. An example of why life-cycle assessment is essential is the case of an automobile using a new fuel that permits the automobile to consume less fuel and emit less CO2 per kilometer traveled while on the road. But there may be no net benefit if more energy and more CO2 emissions are required to manufacture that new fuel instead of the established fuel before fuel ever gets into the automobile tank. The key steps in the life cycle are shown in Figure ES-1.
Primary energy sources such as petroleum or natural gas are considered from the point of their recovery from underground resources through transportation to refineries or manufacturing plants where those sources are converted to fuels for vehicles. The fuel must then be distributed up to deposit in the vehicles tank. The total of these steps is defined here as the fuel cycle or well-to-tank. Analogously, the vehicle cycle begins with ores or other raw materials necessary to make the parts included in a vehicle, fabrication and assembly of those parts, and distribution of the finished vehicle to the customer. The vehicle is then operated by the first or subsequent customer, with maintenance and repair requirements, until the end of its lifetime when the vehicle is scrapped and recycled.
The characteristics of each technology are categorized here as (a) cost, or price, characteristics, (b) environmental, health, and safety characteristics, or (c) other often less- quantifiable matters such as performance, drivability, convenience, reliability, or familiarity. Since each technology includes both fuel and vehicle components, a complete inventory of characteristics must include all the characteristics of fuel and vehicle components of that technology for all the steps shown in Figure ES-1.
The stakeholder groups of concern here (shown in rectangles in Figure ES-1) include the six major groups whose buy-in is required for successful development, introduction, and penetration of a new technology. Those groups include (a) fuel manufacturers,( b) fuel distributors, (c) vehicle manufacturers (including materials and parts), (d) vehicle distributors (including maintenance and repairs), (e) customers for vehicles and fuels, and (f) governments at all levels whose cognizance covers environmental, safety, zoning, and other aspects of new technologies including promoting their development. A complete assessment should consider the impact of each characteristic of each technology on each of these groups; a change that may be trivial to one group may be critical to another group.
An important objective in developing and describing our methodology was to make it transparent and usable by other analysts. Other analysts then have the opportunity to calculate the consequences of assumptions other than the ones we used, and can make use of new information in the future as technologies develop.
Our calculations of the cost, energy consumption, and GHG emissions associated with the production and distribution of each fuel were based primarily on published data. Estimates of future costs included ranges reflecting uncertainties about future prices of petroleum and natural gas and about capital costs of new technologies. Published data were also used to calculate the characteristics of producing, fabricating, and assembling the materials and parts making up the vehicles. The design criteria, performance, and costs of new vehicles were calculated using computer simulations updated and expanded by MIT, and based on previous work at ETH (Eidgenossiche Technische Hochschule) in Zurich; our calculations reflect optimistic but plausible projections of future technologies. The characteristics of all three phases of each life cycle were then combined to make valid integrated comparisons of the technologies assessed.
Scope
The methodology described above was used to characterize technologies with various combinations of the following fuels and vehicle technologies:
- Fuels:
- Gasoline from petroleum
- Diesel fuel from petroleum
- Fischer-Tropsch synthetic diesel from remote natural gas (F-T diesel)
- Methanol from remote natural gas
- Compressed domestic natural gas (CNG)
- Hydrogen from domestic natural gas
- Electric power from the US grid mix of primary energies
- Vehicle Propulsion System:
- Spark ignition internal combustion engines (SI-ICE)
- Compression ignition (diesel) internal combustion engines (CI-ICE)
- ICE-hybrids (combined ICE and battery power plants)
- Fuel cell (FC) hybrids (combined FC and battery power plants)
- Battery-powered electric vehicles
- Other Vehicle Components:
- Automatic, mechanical, continuously variable, and electric drive transmissions
- Evolutionary chassis-body and advanced lightweight designs
Limitations
The most important limitations of our assessment to date are the following:
- We have not considered the often-crucial problems of transition from current to new technologies. We recognize and discuss the transition barriers, conspicuously in introducing new fuels, but have assumed that the barriers have been largely dealt with by 2020 and that new quasi-steady states exist.
- Our analysis is confined to mid-size passenger cars with comparable consumer performance (such as range, acceleration, passenger and cargo capacity) for all technologies. Results for much smaller or much larger vehicles like SUVs, or for other than standard US driving cycles, may be different although we expect directional trends to be similar.
- We assume that, aided by the introduction of low-sulfur fuels, all technologies will be able to reduce emissions of air pollutants to levels at or below US Federal Tier 2 requirements; therefore, non-GHG emissions have not been considered except for exhaust treatment cost to achieve Tier 2 demands.
- We have evaluated only those fuel and vehicle technologies that we think could be developed and commercialized by 2020 in economically significant quantities assuming aggressive development efforts.
There is considerable uncertainty in both the technical and economic results as a result of uncertainty of price (for petroleum and natural gas, for example) and uncertainty about the pace of technical development especially for young technologies such as fuel cells and new batteries.
Results
The key results of this study compare the different technologies we assessed along three dimensions over the entire life cycle in each case. The comparisons assume similar lifetimes and similar driving distances for all vehicles. The three dimensions are: (1)life-cycle energy use, (2) life-cycle GHG emissions, and (3) consumer cost per unit of distance driven. Consumer costs are calculated for a new car buyer and include all fixed and variable costs including typical US fuel taxes. However, fuel taxes can add as much as 6.5/km for a less- efficient car driven in the high-tax UK compared to as little as 0.6/km for a highly-efficient car driven in the low-tax US.
In each case, the more advanced technologies in 2020 are compared to an evolved baseline. That baseline is a mid-size passenger car, comparable in consumer characteristics to a 1996 reference car, in which fuel consumption and GHG emissions have been reduced by about a third by 2020 through continuing evolutionary improvements in the traditional technologies used now.
Figure ES-2 charts energy use, GHGs, and costs for all the new 2020 technologies relative to the 1996 reference car and the 2020 evolved baseline. (The battery-electric car shown is an exception in that it is not comparable to the other vehicles; its range is about one-third lower than other vehicles.) The bars shown are meant to suggest the range of our uncertainty about the results but, as expected, even the uncertainties are uncertain. We estimate uncertainty at about plus or minus 30% for fuel cell and battery vehicles, 20% for ICE hybrids, and 10% for other vehicle technologies.
Conclusions
The results of this study depend importantly on the methodologies and assumptions we chose. The following broad conclusions are drawn from calculations for specific combinations of technology as used in a mid-size passenger car operated over the standard US urban/highway driving test cycles. All our quantitative results are subject to the uncertainties expected in projecting 20 years into the future, and those uncertainties are larger for rapidly developing technologies like fuel cells and new batteries.
- A valid comparison of future technologies for passenger cars must be based on life cycle analysis for the total system, which includes assessment of fuel and vehicle manufacture and distribution in addition to assessment of vehicle performance on the road.
- Successful development and penetration of new technologies requires acceptance by all major stakeholder groups: private-sector fuel and vehicle suppliers, government bodies at many levels, and ultimate customers for the products and services. Therefore, the economic, environmental, and other characteristics of each technology must be assessed for their potential impacts on each of the stakeholder groups.
- Continued evolution of the traditional gasoline car technology could result in 2020 vehicles that reduce energy consumption and GHG emissions by about one third from comparable current vehicles and at a roughly 5% increase in car cost. This evolved baseline vehicle system is the one against which new 2020 technologies should be compared.
- More advanced technologies for propulsion systems and other vehicle components could yield additional reductions in life cycle GHG emissions (up to about 50% lower than the evolved baseline vehicle) at increased vehicle purchase and use costs (up to about 20% greater than the evolved baseline vehicle).
- Vehicles with hybrid propulsion systems using either ICE or fuel cell power plants are the most efficient and lowest-emitting technologies assessed. In general, ICE hybrids appear to have advantages over fuel cell hybrids with respect to life cycle GHG emissions, energy efficiency, and vehicle cost, but the differences are within the uncertainties of our results and depend on the source of fuel energy.
- If automobile systems with drastically lower GHG emissions are required in the very long run future (perhaps in 30 to 50 years or more), hydrogen and electrical energy are the only identified options for fuels, but only if both are produced from non- fossil sources of primary energy (such as nuclear or solar) or from fossil primary energy with carbon sequestration.
Again, these conclusions are based on our assessment of representative future technologies, with vehicle attributes held at todays levels. The expectations and choices of customers may change over the next 20 years and such changes can affect the extent to which potential reductions in GHG emissions are realized.